I'm working on various experiments to answer the fundamental question, what is gravity?
Gravity is a force we experience every day, but its behavior is surprisingly puzzling in many ways. For example, gravity is somehow exceptionally weak compared with other fundamental forces, and it somehow affect all objects equally. The standard model of particle physics can explain all the other interactions such as electromagnetic force, strong force, and weak force, based on quantum mechanics. However, ravity is described only by general relativity. Mysteries in gravity are related to other mysteries such as cosmic inflation, the accelerating expansion of the universe, and dark matter. These mysteries suggest the existence of new laws of physics beyond our understanding of the universe.
To test alternative theories of gravity and cosmology by gravitational wave observations, I'm working on the development of gravitational wave telescopes. Using the interferometer techniques developed for gravitational wave detection, I'm also working on experiments to probe gravity with various approaches, such as dark matter searches, tests of quantum nature of gravity, and tests of relativity.
Gravitational Waves
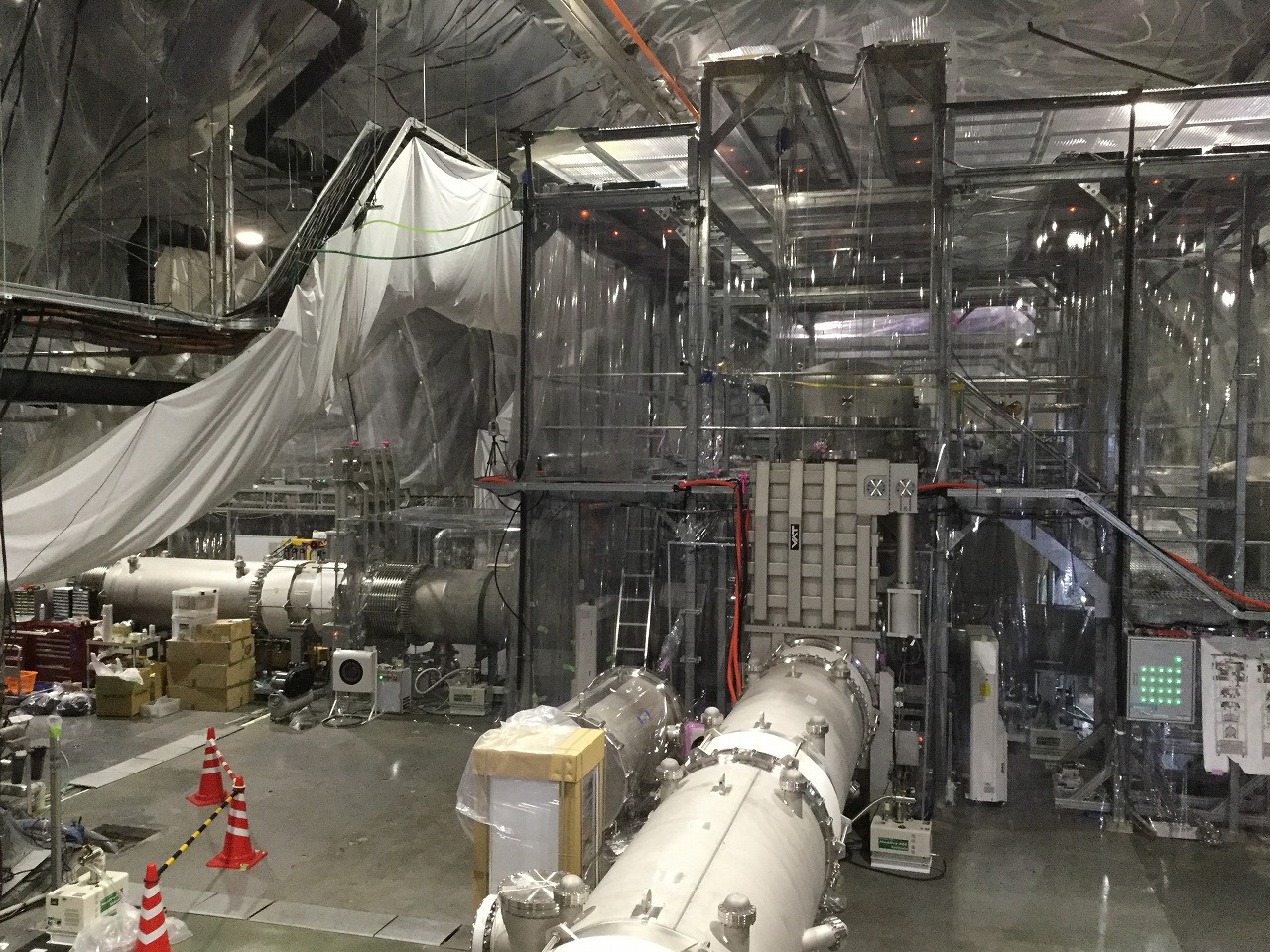
Gravitational waves are ripples in spacetime. Since Einstein's prediction over a century ago, many have attempted to detect these waves but were unsuccessful due to their incredibly subtle effects.
In 2015, the two detectors of Advanced LIGO in the United States made the first-ever observation of gravitational waves, originating from the merger of black holes each with around 30 solar masses. In 2017, with the addition of Advanced Virgo in Italy, gravitational waves from a binary neutron star merger were detected, leading to coordinated follow-up observations by observatories worldwide. These marked the beginning of a new era in gravitational wave astronomy.
To advance the field of gravitational wave astronomy, we need more and better signals from multiple detectors. In Japan, the construction and the commissioning of the gravitational wave telescope KAGRA is underway. KAGRA is the only interferometer in the world which was built in a quiet underground site, and is the only interferometer which will cool down the mirrors to reduce thermal disturbances. As the chair of the Main Interferometer group of KAGRA, I have led the research and development of the interferometer. Additionally, I have been working on quantum noise reduction experiments and researches to explore new materials for mirrors for future upgrade of the detectors.
My research also involves the development of low frequency gravitational wave telescopes. In particular, the space-based detector DECIGO is designed to target the 0.1-10 Hz range, enabling the detection of primordial gravitational waves from the inflationary period. My ultimate goal is to reveal the mechanism of cosmic inflation by the observation of these primordial gravitational waves.
Dark matter search
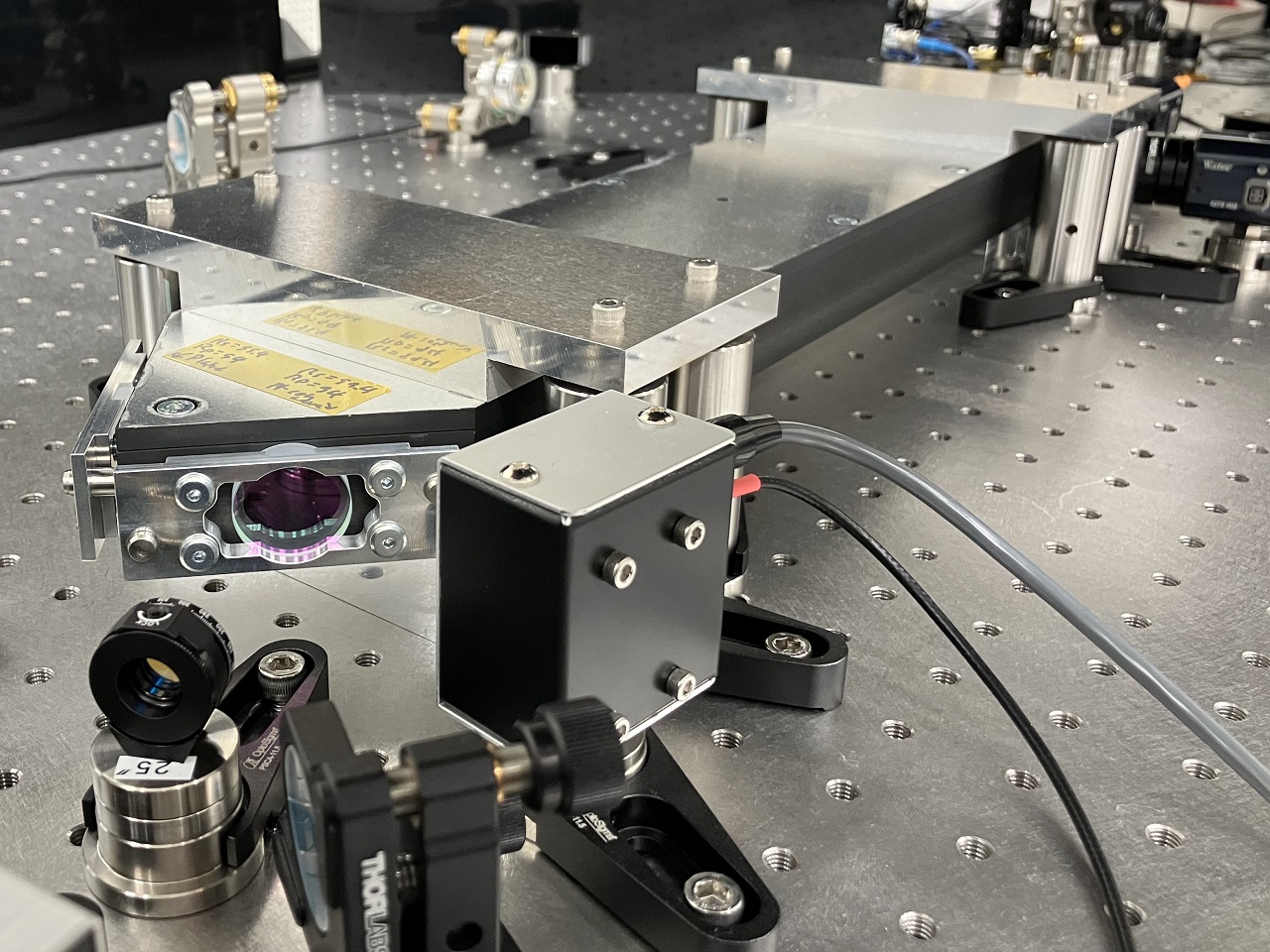
Various astrophysical observations have revealed that our universe is filled with dark matter. Dark matter is an invisible mass that interacts with ordinary matter almost exclusively through gravity. Its existence is strongly implied from observations such as rotation velocity of galaxies and gravitational lensing. Recent observations of temperature fluctuations in the cosmic microwave background revealed that about 80% of whole matter in our universe is dark matter. The standard model of cosmology, based on these findings, is amazingly consistent with other observations.
That said, the nature of dark matter remains unidentified. Especially, WIMPs (Weakly Interacting Massive Particles) and primordial black holes have long been considered as promising candidates. However, after years of large-scale observations and experiments, no definitive detection has been made. This has sparked a growing interest in exploring a wider range of dark matter candidates through innovative approaches.
We are performing experiments to search for ultralight dark matter by investigating its subtle interactions with laser interferometers. Especially, we have proposed a novel method to search for axion dark matter by searching for polarization modulation in laser beams. Ultralight dark matter search can also be done by searching for non-standard forces acting on mirrors of interferometers. With these new methods, we are aiming to search for dark matter with unprecedented sensitivity.
Tests of quantum nature of gravity
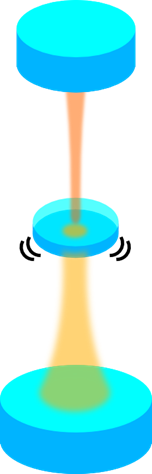
Quantum mechanics fundamentally allows for the superposition of states. Schrodinger's cat, a erll known thought experiment, illustrates this concept with a cat that is simultaneously alive and dead. While quantum superpositions have been observed at the microscopic scale, such as in atoms and molecules, they have never been observed at the macroscopic scale, like in cats. Since quantum mechanics is theoretically scale-independent, we should expect to observe quantum superpositions at the macroscopic scale if the theory is entirely accurate.
This problem has been at the heart of profound mysteries in present physics. Especially, a superposition of position states, such as a mass being left and right, implies a superposition of its gravitational field, and this problem is closely related to quantization of gravity. We might simply don't have enough technology to observe such superposition at macroscopic scales, but on the other hand, it is well known that quantization of gravity is theoretically difficult. There are suggestions that gravity might play a role in destroying macroscopic quantum superposition states.
Recent advances in precision measurements have enabled us to experimentally explore this problem. We are particularly focused on using optomechanical systems to explore quantum nature of gravity. At macroscopic scales, environmental disturbances typically obscure quantum effects. To address this, we proposed a novel method to isolate a milligram-scale mirror by levitating it using the radiation pressure of a laser beam. This optical levitation offers a promising approach to testing the quantum nature of gravity at previously unexplored mass scales.
Lorentz violation search
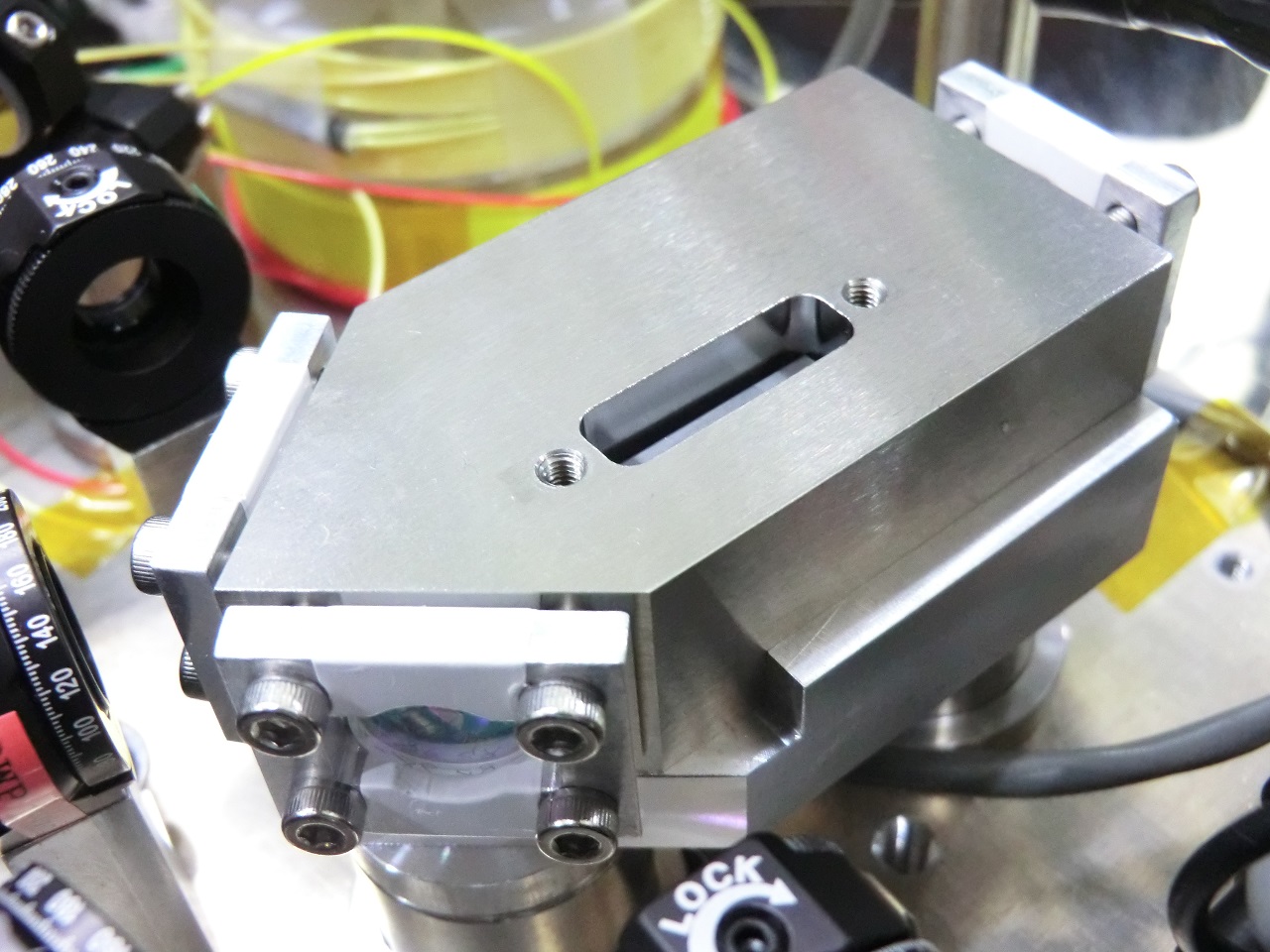
According to Einstein's special relativity, the speed of light is isotropic, meaning it is independent on the direction of propagation. The idea that the laws of physics are independent of the direction is called Lorentz invariance. A wide variety of precise experimental tests of Lorentz invariance have been carried out, with no violations observed to date. As a consequence, Lorentz invariance underlies all the theories of fundamental physics.
However, theoretical works towards the unification of gravity and quantum theory have suggested the possibility of Lorentz violation. If Lorentz invariance were to be violated, the speed of light might exhibit slight anisotropy.
We are performing searches for anisotropy in the speed of light. Especially, we are focusing on measuring the difference between the speed of light propagating in opposite directions, which is difficult to measure with conventional interferometers. We have developed a new type of optical ring cavity to measure the difference. So far, we have succeeded in showing that the relative difference is less than 10-15 level, setting the world best limit. Upgrade of the apparatus is ongoing to perform searches with increasing precision.